BIOCHEMISTRY TOPICS
Thioesters
Role of coenzyme A and other thiol groups in formation of thioesters. Formation of thioesters.
The chemistry of the acyl group and its various derivatives occupy a prominent place in organic chemistry, and it is likewise important in metabolic reactions. In contrast to the various acyl derivatives employed in organic synthesis, one key derivative - the thioester - plays a disproportionately large role in metabolism. The most common thioesters are based on phosphopantetheine (which is the biochemically active form of the pantothenate), which includes coenzyme A ("A" for acylation).
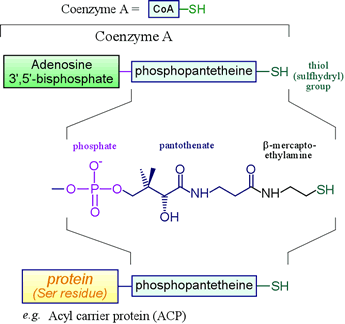
Certain biomolecules serve as carriers of acyl groups. Coenzyme A falls into this category, as does acyl carrier protein, a small protein that participates in fatty acid synthesis by helping shuttle the growing acyl chain between enzymatic active sites. Non-ribosomal protein synthesis also entails amino acyl thioester formation.
Left: The biochemically important thiols based on phosphopantetheine include coenzyme A (CoA) and acyl carrier protein (ACP). The thioesters formed by combination of these thiols with species bearing acyl groups - such as fatty acids - have prominent roles in metabolism. Phosphopantetheine is the combination of 2-mercaptoethylamine, pantothenate, and one phosphate group. Pantothenate is a vitamin - it must be included in our diet or enough acquired through the synthetic activity of intestinal flora.
Thioesters typically arise from or are interconverted with an acyl phosphate species, or an acyl adenylate. Both the latter have greater biochemical standard free energy for acyl group transfer, so ATP can be used to first phosphorylate or adenylate a carboxylate group when net production of a thioester is called for. The acyl phosphate reaction is a means of generating a thioester. The reaction works in reverse as well - the energy of a thioester bond (ΔG°′ = −7.5 kcal/mol for hydrolysis) can be conserved in the terminal phosphoanhydride bond of ATP.
Other types of thioesters that occur in biochemical processes are indicated. A thioester directly linked to a protein via a cysteine residue is generated in the course of the reaction catalyzed by glyceraldehyde 3-phosphate dehydrogenase (GAPDH), an enzyme of glycolysis.
Formation of thioesters
The conversion of a carboxyl group to a thioester occurs in biochemical reactions by four main routes:
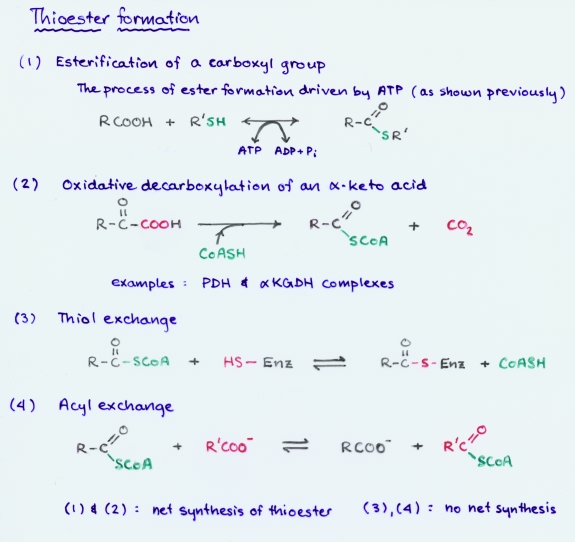
(1) Esterification of a carboxyl group, driven by ATP hydrolysis (as shown above)
(2) Oxidative decarboxylation of an α-keto acid.
There are two closely related examples of this type of conversion, namely in the reactions catalyzed by the pyruvate dehydrogenase complex and the α-ketoglutarate dehydrogenase complex (part of the citric acid cycle
(3) Thiol exchange
(4) Exchange of the acyl group
(1) and (2) result in the net synthesis of a thioester, while in (3) and (4), there is no net synthesis of thioester, but merely exchange of groups.
Reactions of thioesters
Thioesters participate in a variety of reactions. The mechanisms feature attack at the carbonyl carbon to produce a tetrahedral transition state, which can lead to thiol exchange or conversion to acyl phosphate; conjugate addition to an α, β-unsaturated thioester; reactions at the α carbon, such as base-catalyzed enolization, followed by attack of the enol on another carbonyl carbon, as in the formation of acetoacetate or citrate.
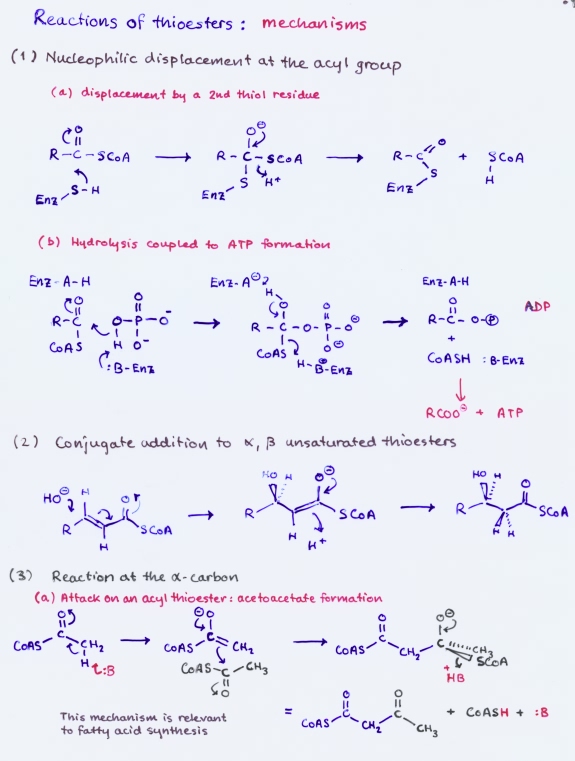
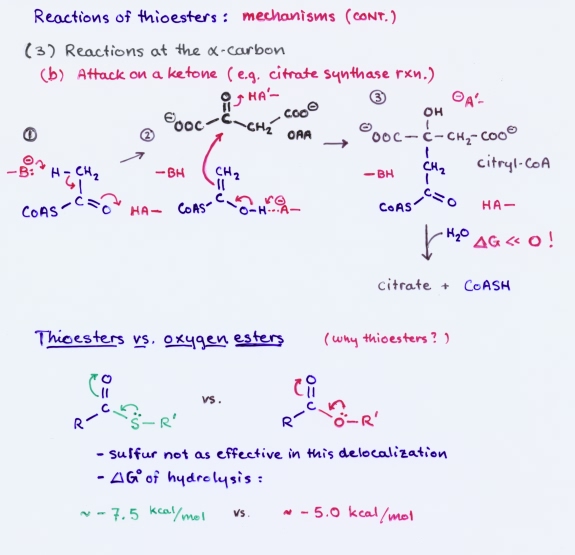
Thioesters play a prominent role in metabolism. This is especially true of fatty acid metabolism. The central metabolite acetyl CoA is a thioester that is produced mainly by oxidative decarboxylation of pyruvate or by fatty acid degradation. The primary fates of acetyl CoA are to enter the citric acid cycle or to contribute to fatty acid synthesis.
Why thioesters?
Given their occurrence in a variety of reactions,
the question may arise concerning the apparent preference in metabolism for thioesters.
The examples above suggest that
(1) Thioesters are effective acylating agents.
(2) Thioesters are also good alkylating agents.
Why are thioesters particularly suited for these metabolic roles
compared to "regular" (oxygen) esters?
We seek a thermodynamic and kinetic account for this observation.
Thermodynamically, the thioester linkage releases more free energy upon hydrolysis
than an oxygen ester.
Kinetically, the reactivity of a thioester at the
alpha carbon compares favorably with that of ketones.
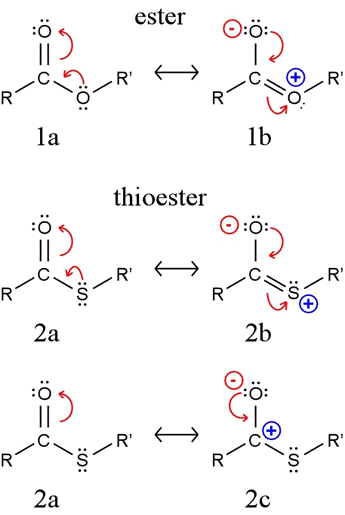
Thioesters as acylating agents: An acylating agent is a molecule that can transfer an acyl group (RCO) to another species. For example, the transfer of an acyl group from acetyl CoA to water produces acetic acid (or more properly, its conjugate base acetate, which predominates at neutral pH). This is simply the hydrolysis of acetyl CoA, and such a hydrolysis has a relatively large negative biochemical standard free energy change (ΔG°′) associated with it: ΔG°′ = −7.5 kcal/mol (−31.4 kJ/mol), which is even slightly more exergonic than hydrolysis of the terminal phosphate of ATP. Thus, just as we say that ATP has relatively high phosphoryl transfer potential, so too does an acyl CoA have a relatively high acyl group transfer potential. By comparison, the ΔG°′ for hydrolysis of a typical ester is about −5 kcal/mol (−21 kJ/mol).
What explains this high acyl transfer potential? An ester (1a in figure) is stabilized by a contribution from resonance structure 1b. A similar structure 2b for a thioester cannot make as much of a contribution because the required overlap between carbon's 2p orbital and the sulfur 3p orbital is ineffective. The large difference in energy between these two orbitals means they cannot "mix" as well as the 2p orbitals of oxygen and carbon.
Because resonance structure 2c makes a more significant contribution for a thioester than an analogous structure for an ester, the acyl carbon is more positive, hence more susceptible to nucleophilic attack. An attacking nucleophile would be more readily acylated by a thioester than it would be by an ester. Furthermore, the C-S bond is weaker than the C-O bond, and the thiolate (or thiol, if protonated) is a better leaving group than alkoxide (or alcohol). These factors all make a thioester in general a better acylating agent than an ester.
One case in which oxygen esters do have a key part in the life of the cell is in the reactions of protein synthesis occurring in ribosomes. The amino acid is esterified to the 3' OH at the 3' end of tRNA to form an aminoacyl-tRNA. This reaction occurs at the expense of hydrolysis of two phosphodiester bonds. Given what is stated above about the energy of hydrolysis of an ester, does this make sense? It does, once account is taken of the special properties of the ester of an amino acid. The inductive effect of the positively charged (at pH 7) amino group destabilizes the reactant ester while stabilizing the carboxylate product of hydrolysis. The standard free energy of hydrolysis of the ester of an amino acid may be more like −8.4 kcal/mol, quite a bit more energetically favorable than hydrolysis of other esters, and representing even more energy than in a thioester of an ordinary carboxylic acid.
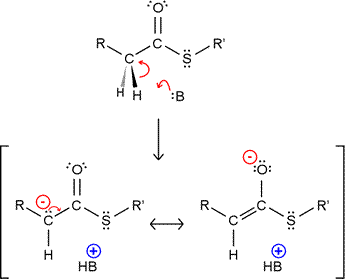
Thioesters as alkylating agents: The stabilization of the resulting carbanion by resonance makes hydrogen attached to the α-carbon of a thioester more acidic. The enolate form (resonance structure on the right in the figure) likely predominates, but the overall effect is to make the thioester more nucleophilic, and thus able to act as an alkylating agent. Nucleophilic attack by the enolate on an electrophilic carbon center, such as a carbonyl or acyl carbon, forms a new carbon-carbon bond. A nice illustration of both of these types of reaction is provided by the formation of ketone bodies. The enolate form of acetyl CoA condenses with another acetyl CoA to form acetoacetyl CoA. HMG-CoA can form by addition of another acetyl CoA in enolate form to the ketone carbonyl carbon of acetoacetyl CoA.