GENERAL CHEMISTRY TOPICS
Lewis structures
Examples of how to draw Lewis structures: Water (H2O), Dinitrogen monoxide (Nitrous oxide, N2O), acetic acid (C2H4O2). General rules for drawing Lewis structures.
Lewis structures are structural formulas for molecules and polyatomic ions that represent all valence electrons. Since valence electrons are typically represented as dots, these structural formulas sometimes are called Lewis dot structures. These symbolic representations were introduced by Gilbert Newton Lewis (1875-1946), a prolific American chemist who was a pioneer in the theory of chemical bonding, thermodynamics, and other areas of chemistry. Lewis structures are of great utility as a tool that allows us to speak a "language" of chemical bonding and molecular structure. The structures, with their guiding rules (principally the octet rule) provide a basis to predict the chemistry of the elements. In other words, we can make a judgment of whether a particular combination of elements is likely to form a stable molecule or polyatomic ion. The further interpretation of Lewis structures in terms of their implied three-dimensional molecular structures and inferred molecular polarity broadens the scope of their usefulness and importance. Therefore, we will place great emphasis upon developing and practicing the skill of correctly drawing and interpreting Lewis structures.
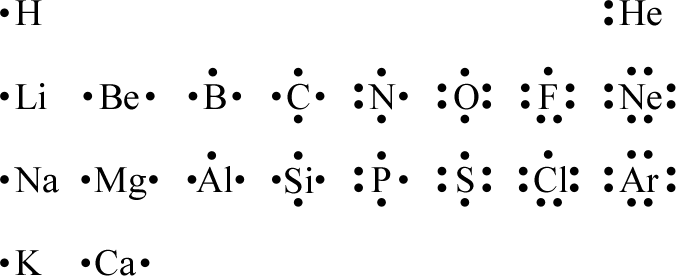
The starting point for Lewis structures are the Lewis symbols for the atoms that comprise the molecular or ionic species under consideration. This is specified by its molecular formula. Recall that a Lewis symbol includes the element symbol plus its valence electrons represented as dots. The Lewis symbols for the first 20 elements are shown in the adjacent figure. From this, we extract what is essential to draw a correct Lewis structure: the element symbol for every atom and a correct total count of valence electrons.
One feature to note about the Lewis symbols above is that they can be seen to represent the periodic properties, which correlate with numbers of valence electrons. The chemistry of the elements with the same number of valence electrons ought to be similar. For the second and third periods, eight valence electrons fills the shell, and the next period begins the next higher shell. This provides the basis for the octet rule.
The next necessary step is to decide how the atoms will be arranged in the Lewis structure, or how all the atoms are linked together by covalent bonds. Our choice for this is called a skeletal structure. For a given chemical formula, it may at first seem that there is more than one way to do this. In many cases, the rules that we will develop presently will render a given arrangement less likely, or very implausible. In other cases, there will be two or more distinct ways of arranging atoms. We denote these as structural isomers, and they are especially common in organic chemistry, which is concerned with molecules largely made up of carbon atoms. The latter are uniquely adept at bonding to each other as well as to a number of other types of atoms.
Now let's start with the simplest molecules and state the rules of the "game" of drawing Lewis structures. A pair of electrons between two atoms will make up a single covalent bond, and by convention we will convert the two dots into a dash to symbolize the bond. For the diatomic hydrogen molecule, H2, there are two total valence electrons, so the steps to its Lewis structure are as illustrated below.

It should be obvious that there are no alternatives to this skeletal structure for H2 and that we have used all available electrons in our Lewis structure representation of the molecule. Our interpretation of it is that diatomic hydrogen should exist as a stable molecule with the two atoms held together by a single covalent bond, i.e. a shared pair of valence electrons localized between them. We know that this "prediction" is true, since the diatomic hydrogen molecule is known to exist as a stable molecule requiring an input of energy to be broken back into two separate hydrogen atoms.
In stark contrast, a similar observation cannot be made for the other Period 1 element, helium. Consider the Lewis structures we might initially propose for diatomic helium:
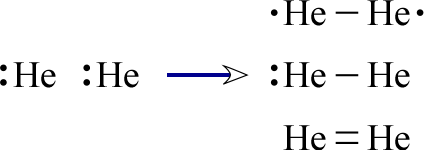
None of the structures we can draw for He2 represent stable molecular forms of helium for the simple reason that helium is extremely unreactive. Any compounds of helium that can be produced not only require significant input of energy but are also quite unstable. Considering the two Period I atoms, the relative stability of H2 and profound instability of He2 suggests that when these smallest of atoms acquire a pair (or dyad) of valence electrons they have achieved a stable electron configuration, which for the helium atom is a filled 1s orbital. Hydrogen does this by forming a covalent bond between two atoms, each atom sharing the pair between them, while helium already is in possession of an electron pair, so has much less tendency to react to form stable compounds.
To summarize thus far, a hydrogen atom can readily form covalent bonds with other hydrogen atoms or (as we'll illustrate below) with other types of atoms, but can accommodate at most two electrons in its valence shell. Therefore, hydrogen atoms can stably form only one covalent bond with another atom. Helium does not form stable covalent bonds.
Other diatomic molecules. Proceeding to Period 2 atoms, but still limiting ourselves to the simplest molecules, starting with the Group 7A (halogen) fluorine, a single covalent bond can be formed between two atoms with each contributing one electron.

This creates a shared pair, or bonding pair, between the two fluorine atoms which completes an octet for both atoms as each also has three unshared pairs (or lone pairs). Indeed, the most stable form of elemental fluorine under normal conditions is as a diatomic molecule, existing in bulk in the gas phase. Furthermore, the other halogens also form diatomic molecules: Cl2, Br2, I2.
The octet rule. The completion of the n = 2 valence shell, as occurs for argon atoms, stabilizes electron configuration and makes argon very unreactive, much like helium. If a similar stabilization occurs for atoms in the context of a molecule, this means that Lewis structures ought to be drawn so that each atom attains an octet (a total of 8) electrons. If this cannot be done with the available valence electrons, one would infer a compound with this elemental composition is unlikely to form. Fluorine and the other halogens in the present example satisfy the octet rule by forming diatomic molecules.
Hydrogen can also combine with any of the halogens to form a series of heterodiatomic molecules, called hydrogen halides. Hydrogen provides the extra electron to give the halide atom its completed octet. We'll revisit other diatomic species, molecular oxygen and nitrogen following a detour into larger molecules.
Triatomic molecules
Once we begin considering molecules with three or more atoms, we are forced to draw skeletal structures with at least one atom that is covalently linked to more than one other atom. For the time being, we'll limit ourselves to structures with just one such atom, called the central atom. The other atoms, bonded only to the central atom are denoted terminal atoms (or peripheral atoms).
Example 1. Water. The formula for
water is H2O.
Counting valence electrons yields eight total (six from oxygen, one each from the two hydrogens).
Hydrogen - as we have seen - is special since it can only accommodate a duet,
and therefore can form at most one bond.
Atoms that can only form one bond must be terminal (or peripheral) atoms in the structure.
The skeletal structure for water must be H-O-H, and not H-H-O, since hydrogen cannot be a central atom.
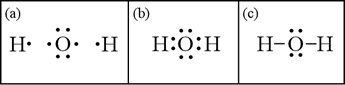
In panel (a), the skeletal structure for water is shown with the atoms represented by their Lewis symbols. The eight total valence electrons are explicitly represented. In (b) we allow single electrons - one each from hydrogen and oxygen - to form a bonding pair between the nuclei. In (c), we have replaced both bonding pairs with a line (or dash) to symbolize the covalent bond formed between the atoms by the bonding electron pair. Note that oxygen is surrounded by an octet of electrons, satisfying the octet rule. We have drawn a valid Lewis structure for water.
Other species closely related to water are the ions hydronium and hydroxide These can be envisioned as arising from addition or removal of H+, respectively.
Example 2. Carbon dioxide.
The formula for carbon dioxide is CO2.
Counting valence electrons yields a total of 16
(12 from the two oxygen atoms with six each, and four from the carbon atom).
Again, there are two possible skeletal structures, O-C-O and C-O-O.
Here the choice will be made on the basis of a general rule -
one we'll use again and explain further -
that less electronegative
atoms tend to be preferred as central atoms.
Furthermore, carbon specifically serves as a better central atom than
any other type of atom due to its characteristic property of forming four covalent bonds
in completing an octet. Therefore, we choose the O-C-O structure.
With two bonding pairs in two single bonds to link the atoms of the skeletal structure,
we're left to fill in the remaining 12 valence electrons so that each atom has a
complete octet, if possible. Carbon dioxide is a stable molecule with this skeletal structure,
so it must be possible!
Once we have a correct count of valence electrons, chosen a skeletal structure, and used pairs of electrons to make single bonds between the atoms of the latter, we'll need to place the rest of the valence electrons. In one systematic method for drawing Lewis structures that applies to this situation, any remaining electrons are distributed as lone pairs to the terminal atoms. Note that we keep electrons always in pairs - as either bonding or nonbonding pairs - which is not a problem if the total number of valence electrons is even (In all the examples we consider here, the number of valence electrons is even. We treat species with odd numbers of electrons separately as one of the exceptions to the octet rule). Once we have distributed all the valence electrons in this manner, we look at our provisional Lewis structure to see if the octet rule is satisfied. Here, since carbon ends up with a deficiency, we convert lone pairs on the terminal oxygen atoms to bonding pairs between oxygen and carbon. Both covalent carbon-oxygen linkages become double bonds, and carbon thereby receives an octet as it normally does, here by forming four covalent bonds with its neighbors. Generating this structure from the arrangement of atoms and the count of valence electrons is illustrated in the left column in the figure below.
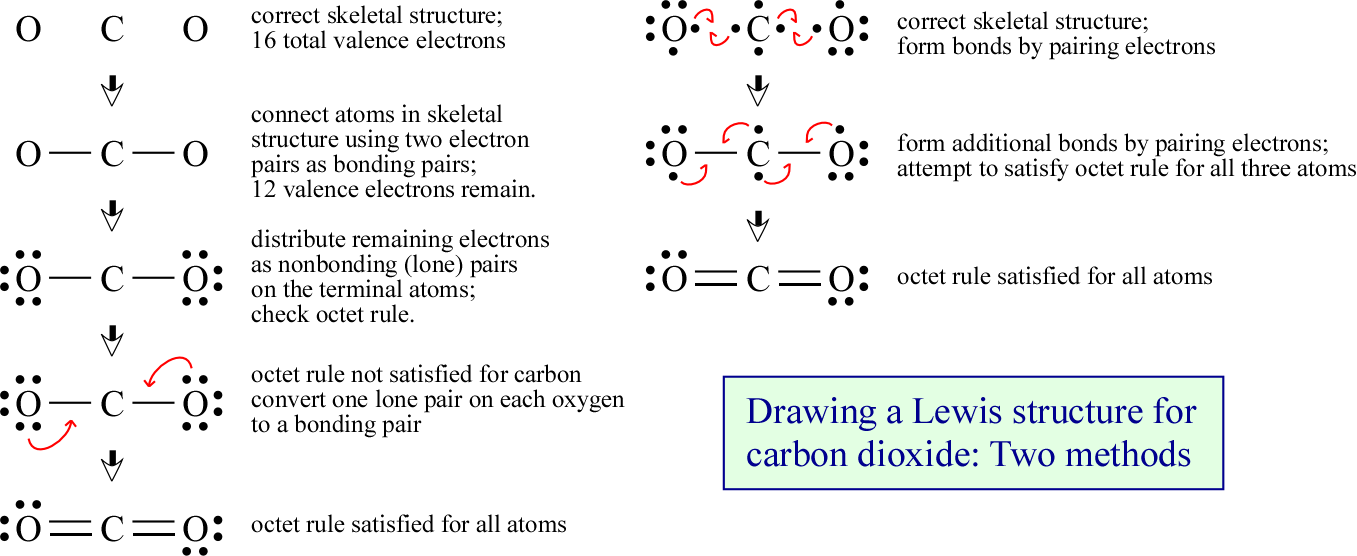
The right column, or second panel in the figure above illustrates the generation of the same structure by starting with arrangement of Lewis symbols according to a chosen skeletal structure, then moving single electrons from the Lewis symbols of adjacent atoms into a shared pair. The shared pairs are bonding pairs, as represented by the dash or line between the atomic symbols. If there are additional single electrons present on adjacent atoms, these are also paired in additional bonds. Double and triple bonds are allowed. From this point the electrons are moved if necessary in pairs in order that the octet rule is satisfied. This method is based on writing the Lewis symbols just as shown in the first figure at the top of the page. The number of single electrons in each case is the same as the number of covalent bonds that the element typically (but not exclusively) forms in molecules. This typical pattern of bonding can be referred to as the normal valence for an atom, "valence" being a general term for number of bonds an atom forms. The valid Lewis structure obtained for CO2 features normal valences for oxygen (2) and carbon (4).
Example 3. Dinitrogen monoxide (Nitrous oxide, N2O).
Since either N or O can serve as a central atom, in choosing a skeletal structure
we again use the guideline that the least electronegative atom be placed in the middle.
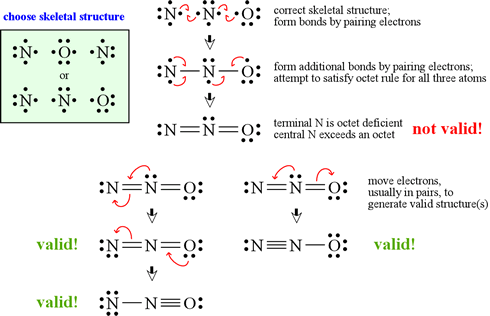
Having the appropriate skeletal structure and correct count of valence electrons, the goal is to place the electrons in bonds or lone pairs so that each atom has an octet of electrons. The figure at left shows a procedure for generating candidate structures by moving electrons singly, or in pairs, starting with the Lewis symbols for the elements. Since N and O are both Period 2 elements, no atom can exceed an octet. In this case, it is possible to draw three valid Lewis structures.
These three valid Lewis structures for dinitrogen monoxide are known as non-equivalent resonance structures. As an assessment tool, formal charge assignments can be used to predict the relative contributions of the resonance forms to the resonance hybrid, which represents a more realistic conception of the electron distribution within the molecule.
Lewis structures with more than one central atom
As we include more and more atoms in a structure, the number of possible skeletal structures begins to grow exponentially. Especially with organic molecules, carbon atoms can constitute links in a chain, or backbone of central atoms.
Example 1. Structural isomers of C2H6O. What distinct skeletal structures can be drawn for a molecule with the formula C2H6O?
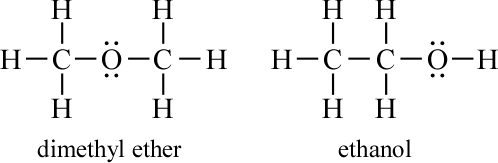
We have two choices for linking the three non-hydrogen atoms as central atoms: C-C-O or C-O-C. A count of valence electrons yields 20, and in both these cases, the completion of the covalent skeleton uses 16 of these 20 electrons. The remaining four electrons are placed on the oxygen atom to complete its octet.
Example 2. Acetic acid (C2H4O2).
There are cases where even for very simple molecules
there are several chemically plausible skeletal structures.
For structures of larger molecules, in which more than one atom is joined to two or more atoms,
there is no longer a unique central atom, and the probability of encountering isomers dramatically increases.
In order to draw the Lewis structure for a given isomer,
more information about the skeletal structure is necessary.
Such is the case for most organic molecules, those containing primarily carbon, hydrogen, and oxygen,
with other elements such as nitrogen, sulfur, and phosphorous also common
in molecules of biological importance.
An example is acetic acid, an important example of a weak acid.
For acetic acid, the skeletal structure is centered on a chain of atoms bonded together as C C O.
The first carbon atom uses its three remaining valence electrons to form bonds to three of the four hydrogens.
To the second carbon atom is attached another oxygen atom.
Finally, the remaining hydrogen atom attaches to one of the oxygen atoms.

In this example, once the skeletal structure is specified, a Lewis structure follows fairly readily. Drawing bonds in place of pairs of electrons composed of one electron from each atom yields a bonded skeletal structure. The octet rule can be satisfied for all non-hydrogen atoms when the remaining unpaired electrons are moved in to form a double bond between carbon and oxygen.
Rules for drawing Lewis structures
Goal: Given a chemical formula corresponding to a molecule or molecular ion, draw a Lewis structure.
1. First of all, a correct count of all valence electrons is essential. One way to do this is to write the Lewis symbols for all of the atoms in the formula, and count up all the "dots". For a molecule (uncharged), that count is the correct number of valence electrons. For polyatomic ions, total the valence electrons for all atoms in the formula and subtract one electron for each positive charge of a cation, and add one electron for each unit negative charge of an anion.
2. Draw a skeletal structure. What this means is that we decide how the atoms are to be bonded. Choose a central atom (we'll start with small molecule examples for which there is only one central atom, and the other atoms - the peripheral atoms - are all bonded to the central atom). Hydrogen (H) and fluorine (F) each have valence of 1, and generally these will not be central atoms (bonded to more than one atom). Given a formula, the central atom is typically the first atom (ClF4 example), although this convention is not always followed (e.g. HNO3). Another good way to choose is to pick the least electronegative atom. Inevitably, there will be cases where it is possible to draw more than one skeletal structure.
3. Draw bonds as lines between atoms. Each bond counts as 2 e−.
4. Add electrons as non-bonding lone pairs around the peripheral atoms so that they have octets (eight electrons total). Note that this does not apply to H, which can only accommodate a duet (2 e−).
5. Add remaining pair(s) of electrons to the central atom so that its octet is complete (if not already). Never exceed an octet for a period 2 atom! For periods 3 and greater, atoms are large enough to accommodate more than an octet in their valence bonding shell. If there are no further electrons available and the central still does not have a complete octet, a lone pair on a peripheral atom may be pushed into a second (or third) bond with the central atom. Carbon and nitrogen are 2nd period elements that commonly form double and triple bonds as central atoms, and oxygen as a peripheral atom is often found in a double bond with the central atom.
6. If all atoms from the 2nd period and greater have at least an octet, and no 2nd period atom exceeds an octet, and the total number of electrons in bonds and lone pairs is equal to the total number of valence electrons available, then a valid Lewis structure has been produced. The convention for ions is to enclose the structure in brackets, and indicate the net charge at the upper right corner.
7. Evaluation, exceptions, and use. Note that there are a few cases where the best Lewis structure has an incomplete octet on a central atom. Since it is often possible to draw more than one valid Lewis structure for a molecule or molecular ion, we will need evaluate which one(s) are more plausible or make better chemical sense. As noted above, formal charge is used as a guide in that total number of formal charges zero or a minimum is generally best, and the formal charge of an atom is considered in relation to its electronegativity. Remember, a Lewis structure is not the molecule, but only a shorthand symbolism that is meant to convey some information about it. Such information informs prediction of the likely physical or chemical properties of the real molecule or the bulk substance that is made up of those molecules. One of the most common uses we make of a valid Lewis structure (and for this we do not need the best Lewis structure, just any valid one) is to predict molecular shape and polarity.
Octet rule
Whenever possible, the valence electrons are distributed in such a way that eight electrons (an octet of electrons) surround each main-group element (except hydrogen, which should have two electrons).
Important auxiliary rule: Never exceed an octet for a Period 2 atom.
Period 2 elements can accommodate at most eight electrons in their valence shells. Beginning with Period 3, this rule is relaxed to explain the existence of molecules with trigonal bipyramidal or octahedral shapes (five or six bonded atoms surrounding a single central atom). For the n = 3 shell and beyond, the number of extra orbitals in higher subshells rapidly expand. For Period 3 elements such as sulfur and phosphorus, the 3d orbitals are accessible to accommodate extra electron groups. These hypervalent molecules are discussed further in the page devoted to exceptions to the octet rule.