BIOCHEMISTRY TOPICS
Carbohydrates
Simple carbohydrates: monosaccharides. Families of aldoses and ketoses based upon D-glyceraldehyde and dihydroxyacetone (respectively). Some important monosaccharides: glucose, fructose, and ribose. Cyclic forms of monosaccharides. Glucopyranose formation. Glycosidic bonds and disaccharides. Complex carbohydrates, glycogen and cellulose. Glycobiology.
Carbohydrates comprise one of the four major classes of biomolecules (along with proteins, nucleic acids, and lipids. Carbohydrates are aptly named as their general elemental composition is reflected by the formula (CH2O)n. More precisely, carbohydrates are or are based upon polyhydroxy aldehydes or ketones. The simplest carbohydrates are the monosaccharides, such as glucose fructose, and ribose. The more complex forms - disaccharides and polysaccharides - are built up as polymers of monosaccharides. Table sugar or sucrose is a disaccharide composed of glucose and fructose. Complex carbohydates include glycogen, a branched polymer of glucose units that is a storage form of carbohydrate in animals, and cellulose, a linear polymer of glucose units that occurs in plant cell walls.
Carbohydrates are the prime example of metabolic "fuel" for cells. The stepwise degradation of the monosaccharide glucose is a nearly universal pathway (see glycolysis) that yields biochemical energy in the form of adenosine triphosphate (ATP). Plants, in carrying out photosynthesis, harvest the energy of sunlight by using it to convert carbon dioxide and water into carbohydrates and oxygen. The so-called "dark" reactions of photosynthesis, known as the Calvin cycle, are responsible for the uptake of carbon dioxide and its assimilation into carbohydrates.
Monosaccharides
Since carbohydrates are aldehydes or ketones with two or more hydroxyl groups, the simplest monosaccharides are the three-carbon compounds glyceraldehyde and dihydroxyacetone (DHA), which are shown in the figure below. Simple carbohydrates with an aldehyde functional group are called aldoses, while those with ketone functional groups are called ketoses. Glyceraldehyde is thus the simplest aldose and dihydroxyacetone is the simplest ketose.
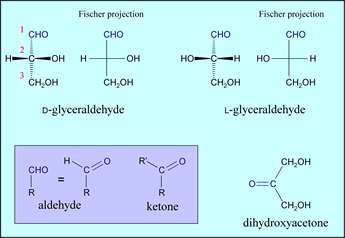
The middle carbon atom (C2) of glyceraldehyde is chiral, since there are four different groups bonded to it. Therefore, there are two enantiomeric (mirror-image) forms of glyceraldehyde, D-glyceraldehyde and L-glyceraldehyde, both of which are shown. (The term "enantiomer" is equivalent to "stereoisomer".) Fischer projections (explained further below) are shown for both stereoisomers. In the case of DHA, the middle carbon corresponds to the carbonyl group. D-glyceraldehyde, L-glyceraldehyde, and dihydroxyacetone are structural isomers, since each has the chemical formula C3H6O3.
Note that the Fischer projections imply a stereochemical convention, as indicated by the side-by-side structural formulas above for both the D and L configurations of glyceraldehyde. At any given chiral carbon, the horizontal bonds are taken to project up out of the plane of the figure, while vertical bonds point back behind the plane. We will use Fischer projections to represent the families of larger (4, 5, and 6 carbon) sugars based on D-glyceraldehyde and DHA.
Both the hydroxyl and carbonyl groups are polar and have the ability to participate in hydrgen bonds (a carbonyl group can only act as an acceptor, however). Consequently, carbohydrates are hydrophilic in character. It is worth noting that many of the properties of carbohydrates relate to the reactive nature of the carbonyl group, common to both aldoses and ketoses.
D-glyceraldehyde and DHA can be considered the parent compounds of a series of monosaccharides with more carbon atoms. Larger monosaccharides based on D-glyceraldehyde can be constructed by inserting extra CH(OH) groups between the aldehyde group (its carbon atom is always designated as C1, and is shown in blue) and the chiral group with the D configuration like C2 of D-glyceraldehyde. This group is always taken to be the one furthest away from C1 (always shown in red in the figure below).
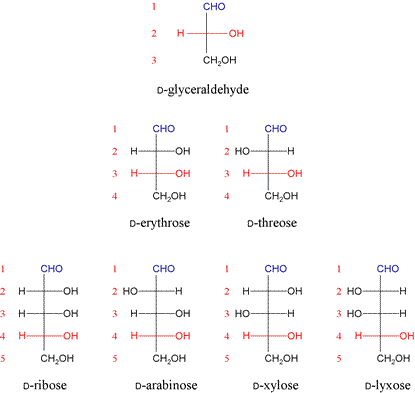
The addition of the first CH(OH) group creates two possible tetroses (monosaccharides containing four carbon atoms) from the parent D-glyceraldehyde (a triose), since added group creates a new chiral center. Note that these tetroses, D-erythrose and D-threose, are not mirror images of one another, but are diastereomers. (Note also the resemblance of D-threose to the amino acid threonine, where C2 of the tetrose corresponds to the alpha carbon of the amino acid.) Another point of terminology that arises in comparing the two tetroses. Monosaccharides differing in configuration at a single chiral carbon are known as epimers. Thus, D-erythrose and D-threose are epimers, differing only in the configuration at C2.
The addition of another CH(OH) group results in four possible diastereomeric pentoses based on D-glyceraldehyde. The next addition of CH(OH) generates the series of eight diastereomeric hexoses based on D-glyceraldehyde.
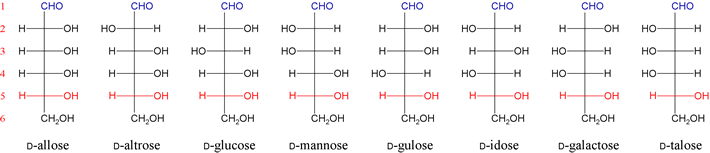
The aldoses encountered most frequently in the study of metabolism are D-glyceraldehyde and its phosphorylated derivatives, as well as D-glucose and derivatives thereof. Ribose and the related deoxyribose are integral components of nucleotides and nucleic acid structure. Mannose and galactose also have relatively significant roles in biochemistry, but other members of this aldose family are rarely encountered.
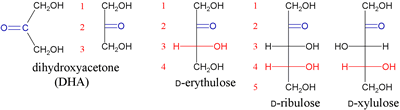
The family of ketoses based on dihydroxyacetone (DHA). Adding an additional H-C-OH group to DHA yields a four-carbon ketose called erythulose.
This additional group confers a chiral center, thus erythulose exists in two enantiomeric forms, D- and L-. Note that the configuration at C3 of D-erythulose matches that of C2 in D-glyceraldehyde. Each subsequent addition of a CH(OH) group to give monosaccharides with one more carbon atom creates an additional chiral center, just as it did in the series of aldoses based on D-glyceraldehyde. Since DHA does not contain a chiral center, there is one less chiral center in the ketoses, for the same number of carbon atoms, compared with the aldose family members. Again, the linear representations of ketoses shown are Fischer projections.
There are two ketopentoses derived from D-erythulose, D-ribulose and D-xyulose. In turn, each of these is the basis for two ketohexoses. D-psicose and D-fructose are based upon D-ribulose. D-sorbose and D-tagatose are based on D-xyulose.
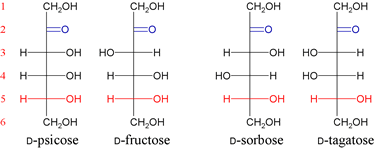
DHA, D-erythulose, D-ribulose and D-xyulose are all biologically relevant. The only important member of the four ketohexoses is D-fructose.
Cyclic forms of monosaccharides
Pentoses and hexoses typically adopt cyclic forms when the open-chain forms isomerize to intramolecular hemiacetals or hemiketals.
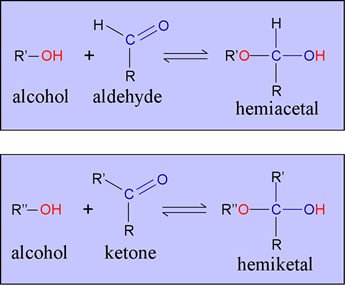
An aldehyde and an alcohol can react to form a hemiacetal. Note the similarity to tetrahedral intermediates we have encountered in reactions of carboxylic acid derivatives such as amides and esters. The tendency for this reaction to occur in a six-carbon aldose is greater because the hydroxyl and aldehyde functional groups are contained within the same molecule, and the intramolecular reaction can produce a relatively stable six-membered ring. The analogous process occurs for ketoses - intramolecular hemiketal formation results in a five- or six-membered ring.
Glucopyranose formation
Cyclization of the open-chain form of D-glucose by intramolecular hemiacetal formation results in a D-glucopyranose molecule.
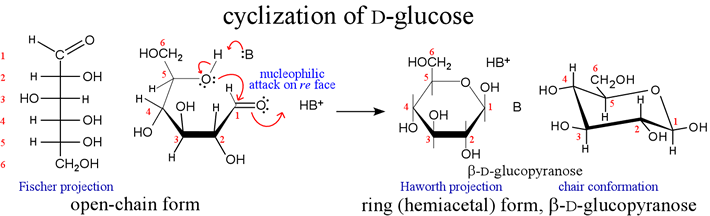
The cyclization creates an extra chiral center at C1 so there are two forms of D-glucopyranose designated α and β (alpha and beta). These alternate forms are called anomers, and C1 is here the anomeric carbon. Note that the anomers can be easily interconverted by reversion to the open-chain form, and recyclization into the other anomer. The representations of the cyclic forms are known as Haworth projections. The thick bonds of the bottom of the ring are meant to suggest the "plane" of the ring projecting toward the viewer, with the bottom of the ring closest.
A drawback of Fischer and Haworth projections is that they do not explicitly show the actual conformation(s), or location of all the atoms of the sugar molecule in three-dimensional space. More stereochemically realistic representations of the conformations of the furanose and pyranose rings common in biochemistry are conveyed by drawings of "envelope", "chair", and "boat" forms as shown below.
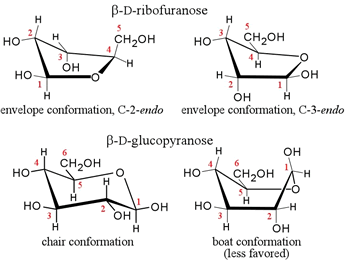
The pentoses ribose and deoxyribose form intramolecular hemiacetals. These furanose rings constitute most of the backbone structure of nucleic acids. For glucose, the chair conformation is the most common pyranose ring conformation, as shown for β-D-glucopyranose (lower left). In all these cases, there is no "plane" of the ring - the ring is puckered. Considering ring substituents, there are two types of positions: equatorial and axial. The former are pointing out further away from the ring and are less sterically constrained. The latter are pointing up or down in the figure, are closer to the bulk of the ring and hence in more "crowded" positions. This is most evident for the chair.
There are many possible conformations for furanose and pyranose rings, but the most stable conformation for a particular sugar depends on the configuration of its chiral carbon atoms, and whether there are any modified substituents. (For example, one or more hydroxyl groups could be involved in ether linkages with another alcoholic (hydroxyl) group, or an ester linkage with a phosphate group.) Chair conformations tend to predominate for most pyranose rings because the steric repulsions are usually minimized. Interestingly, for D-glucose, all the bulkier substituents of the ring (OH groups and the C6 substituent) can occupy equatorial positions in a chair form of a pyranose ring when the anomeric carbon is in the β configuration.
Glycosidic bonds and disaccharides
The more complex forms of carbohydrate are built up as polymers of monosaccharides. Fundamental to the ways in which simple sugars can polymerize is the glycosidic bond. In principle, any of the hydroxyl groups could form, say ether or ester linkages. However, when the hydroxyl group attached to the anomeric carbon condenses with another alcohol, converting the hemiacetal to an acetal, the bond created is called a glycosidic bond, or (specific to this case) an O-glycosidic bond. If the reaction occurs with an amine, an N-glycosidic bond is formed.
Upon formation of a glycosidic bond, mutarotation is blocked in the glycosyl residue, since its anomeric carbon is derivatized. This also protects the monosaccharide unit against oxidation by cuprous ion under basic conditions (Fehling's test), thus the sugar unit is said to be nonreducing.
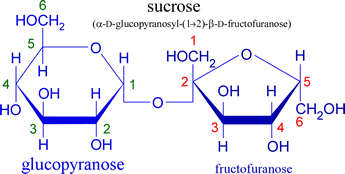
Table sugar or sucrose is a disaccharide composed of glucose and fructose. Note that the glycosidic bond links the anomeric carbon atoms of both sugar units, hence sucrose is nonreducing. Other common disaccharides are maltose and lactose.
Phosphorylated forms of monosaccharides are prominent in biochemistry. A glycosyl phosphate ester is somewhat activated target for a nucleophilic substitution (glycosyl transfer) reaction. An example is glucose 1-phosphate.
Complex carbohydrates
Complex carbohydates include glycogen, a branched polymer of glucose units that is a storage form of carbohydrate in animals, and cellulose, a linear polymer of glucose units that occurs in plant cell walls. Amylose and amylopectin are two forms of starch, polymers of glucose.

Glycobiology
Glycobiology is a relatively new branch of molecular and cell biology that is centered on the role of oligosaccharides (or glycans) as essential biochemical and structural components of eukaryotic cells. For the most part, glycans are attached to macromolecules at the surface of cells, and form a dense layer called the glycocalyx. Cell surface glycoproteins play important mediating roles in cell-matrix and cell-cell interactions. These roles are further elaborated in the context of complex multicellular organisms, where glycans participate in tissue and organ development and structure, and are involved in molecular mechanisms of pathogenesis, such as those occurring when a parasite gains access to a host.
The types of monosaccharide units common in animal glycans are sialic acids, hexoses, hexosamines, deoxyhexoses, pentoses, and uronic acids (Ref 2, §64). The term glycoconjugate is used to refer in general to glycans or oligosaccharides attached to another class of biological molecules, forming glycolipids and glycoproteins.
For more about glycobiology, see Ch.11 of Ref.1, starting at the bottom of p.312, and Ref.2 for a more comprehensive view.
Related topics pages:
- proteins
- nucleic acids
- lipids
- glycolysis - metabolism of glucose
- water