BIOCHEMISTRY TOPICS
Water
Water 101. Further implications of water as the biological solvent.
Water (H2O) is a familiar yet fascinating substance. It provides us with an opportunity to consider the properties of three phases of matter - the gas, liquid, and solid phases - using a familiar and important example. Water is a both an acid and a base, and is an effective catalyst for proton transfer reactions. Water is central to life and the biochemical processes taking place in living organisms. With the majority of the earth's surface covered with water, its properties have a huge impact upon the planetary environment permitting and sustaining the development of the biosphere.
Structure of the water molecule
To start with, let us generate a Lewis structure for the water molecule based on its molecular formula, H2O. The Lewis symbols for the elements are shown in panel (a) below: Oxygen has six valence electrons, and hydrogen only one.
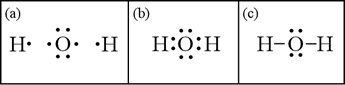
Panel (b) shows how to put the three atoms together to form a complete octet for the central oxygen atom. Hydrogen, as a period 1 element, can only accommodate two electrons in its valence shell, and forms only one bond. In panel (c), we have applied the symbolic convention that two shared electrons that form a chemical bond between atoms can be represented by a line. With a valid Lewis structure, we can infer molecular geometry of the water molecule. The octet about the central oxygen can be viewed as being made up of four electron domains, two bonding pairs and two non-bonding "lone pairs". Application of VSEPR theory to this configuration leads to the conclusion that these four electron domains are - to a first approximation - arrayed about the central oxygen in a tetrahedral pattern. The main conclusion that follows is that the arrangement of atoms in the H-O-H molecule is not linear, but bent, with the bond angle expected to be close to the tetrahedral value, 109.5°. Since the lone pairs spread negative charge over a greater volume than bonding pairs, electrostatic repulsion drives the bonding pairs a little closer together, so the actual bond angle should be a little less than 109.5°. As we'll show below, we can also use the Lewis structure to analyze whether the water molecule has polarity.
What we refer to as "molecular shape" is what we see when we look only at the atoms that make up the molecule. Experimental techniques such as X-ray crystallography that can locate the positions of the atoms in molecules reveal the shape of the water molecule. The diagrams below represent the experimentally determined molecular shape.
In agreement with our analysis using the Lewis formalism, water's shape is angular, or bent, with an H-O-H bond angle of 104.5°. This is consistent with a roughly tetrahedral orientation of four electron domains about the central oxygen, two bonding pairs and two non-bonding "lone pairs".
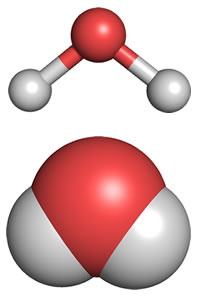
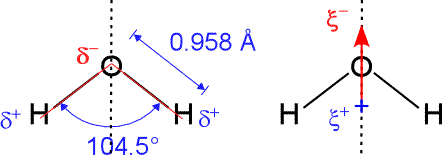
The H-O bond length is 0.958 Å (1 Å = 10−10 m). Since oxygen is significantly more electronegative than hydrogen, more of the negative charge of the electrons in the bonding pair is concentrated near the oxygen. This small separation of charge along an individual bond axis (symbolized by the δ notation) creates a bond dipole. The bond dipoles can be treated as vectors, and summed to yield another vector, the molecular dipole moment.
Above: two representations of the molecular shape of a water molecule, ball-and-stick (top) and space-filling (bottom). Oxygen is the central atom, shown in red, and the hydrogen atoms are in light gray.
If a molecule has a non-zero dipole moment. it is said to be polar, or to have polarity. For the geometry of the water molecule, the bond dipole components parallel to the line bisecting the H-O-H bond angle (dashed vertical line in figure) add together, while the perpendicular (horizontal) components cancel out. This results in a net dipole moment aligned with the bond angle bisector, with negative end pointing up (arrow in right-hand panel above). Thus, the water molecule has a significant dipole moment, which can be modeled as a vertically oriented partial charge separation (labeled as ξ in the figure). In other words, the water molecule is polar.
If shown in these representation, the lone pairs would project upward from the oxygen forward out of the plane and backward behind the plane of the figure (see the next figure below).
Properties of water
Our consideration of the properties of water can serve as a paradigm for the pursuit of one of the most fundamental goals in the study of chemistry. That is to answer the question: How do the nanoscale, molecular properties of water account for the properties we observe of the substance in bulk, at the macroscopic scale? To address this question, we'll make use of the "enhanced" version of the Lewis structure for the water molecule shown below, at right.
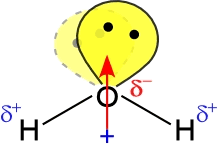
Given a structural interpretation of the water molecule, what intermolecular forces would we predict to be important in determining its bulk properties? The diagram at right shows the geometry of a water molecule, the polarity of its bonds, the molecular dipole moment, and the orientation of lone pairs. Water is not only a polar molecule - it can also form intermolecular hydrogen bonds (H-bonds). The relatively strong intermolecular forces resulting from dipole-dipole and H-bond interactions account for properties such as its high boiling point for a small molecule, a high enthalpy of vaporization, large heat capacity, and an ability to dissolve polar and many ionic compounds.
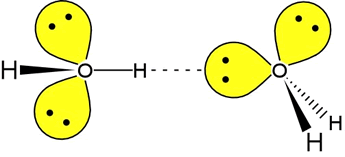
In fact, water is a paragon in the realm of hydrogen-bonding molecules. Each water molecule has two lone pairs to serve as hydrogen bond acceptors, while the two O-H bonds provide a pair of hydrogen bond donors. In the figure at left, one H-bond between two water molecules is depicted. The leftmost water molecule is the H-bond donor, and the water molecule on the right is the H-bond acceptor.
Structure of ice
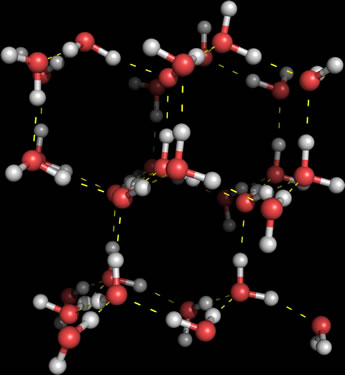
The structure of ice shows the capacity of water molecules to form intermolecular hydrogen bonds, or H-bonds (indicated by the dashed lines in the figure at right) at its maximum. Each water molecule internal to the lattice (that is, not located on the surface) makes hydrogen bonds with four neighbors, accepting two H-bond donors at its central oxygen atom, and donating two H-bonds via its two hydrogen atoms. Thus, each interior water is fully hydrogen bonded, and the overall structure forms a three-dimensional hexagonal lattice of water molecules. The extensive hydrogen bonding lends strength to the ice lattice, but at the same time yields a relatively open structure. This accounts for the fact that at or near the melting temperature, liquid water has a greater density than ice. When the structure of ice melts, some of the hydrogen bonds are disrupted, and the less rigidly constrained water molecules can approach one another a little more closely on average.
Water as a solvent
The ability of water to act as a solvent is related to the polarity of the water molecule, as well as its ability to form hydrogen bonds with solute species. As we have seen, the water molecule is polar, and its dipole moment can be represented as a vector that bisects the H-O-H bond angle. The dipole moment of a molecule acts analogously to a bar magnet in a magnetic field. The negative end of the dipole will tend to align toward positively-charged species, while the presence of a negative charge will attract the positive end of the dipole. Aligning a dipole with the local electric field in this way lowers electrostatic potential energy, making alignment favored.
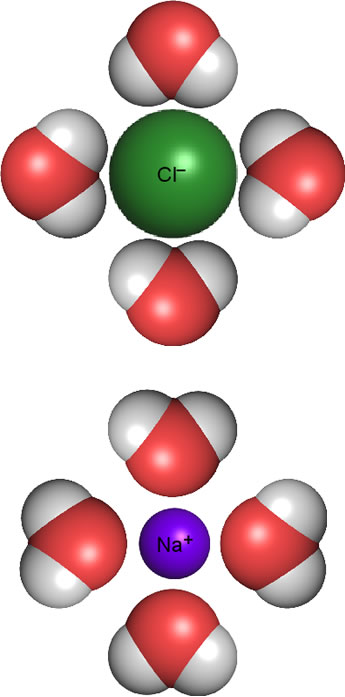
The figure at right shows how this works for ions resulting from sodium chloride (NaCl) dissolved in water. Upon dissolving, the ions making up the salt dissociate from the solid crystalline lattice. The favorable electrostatic interactions of ionic bonds of the lattice are lost, raising potential energy, but the dipole-ion interactions help make up for this. The water molecules near ions orient their dipole moments according to the ionic charges - positive ends of their dipoles toward chloride anion; the negative ends of the water molecule dipole point toward sodium cations. This is referred to as hydration or aquation, and the water molecules involved are called waters of hydration (or aquation).
Whether or not a given ionic compound is soluble in water is in large part determined by the energetic balance between the ionic bonds in the solid state (plus the H-bonds between water molecules in the liquid state) versus the ion-dipole interactions between solute and solvent species in solution. We know that not every ionic compound is freely soluble in water, and we can rely on empirical solubility rules to guide us in answering the question of whether a given ionic compound will be soluble in water. But the considerations above about the energetics of ionic bonding and nonbonding interactions largely determine the solubility rules. Furthermore, compared to a less polar solvent (such as methanol) or a nonploar solvent (such as hexane or benzene), water is a much more effective solvent for ionic compounds. Nonpolar or weakly polar solvents are not nearly as effective as water in solvation of dissociated ions, thus we have no need for solubility rules for ionic compounds in such solvents - we would uniformly predict ionic compounds to be insoluble in nonpolar solvents.
For molecular compounds, water is generally an excellent solvent for those with polar molecules, and even more so for polar molecules with ability to form H-bonds with water molecules.
Water and life
Although they are quite complex and heterogeneous in structure and composition, living cells are largely aqueous systems. The fact that water is mostly a liquid within the broad range of temperatures typical of the earth's surface and its ability to dissolve a wide variety of compounds makes the existence of such complex systems possible. Water can justifiably be called the solvent of life. Certainly if we are to understand biological chemistry, we would do well to study carefully the properties of water.
Often cited in this regard is water's unusual characteristic of forming a solid with a lower density than the liquid state. The relatively open structure of ice maximizes the hydrogen bonding capacity of the constituent water molecules, making this open structure energetically favorable. When ice melts, some of the hydrogen bonds are broken, which allows water molecules to approach each other a little more closely on average. Thus liquid water, in equilibrium with ice at 0°C, is more dense than the ice, which floats. Water is at maximum density in its liquid form at 4°C. This has tremendous consequences for the earth's biosphere. Lakes and oceans would freeze from the bottom up if ice were more dense than water. This would result in most of the water on earth being locked up in the form of ice.
Of special note among the chemical propeties of water is that it is both a very weak acid and a very weak base, and proton-transfer reactions occur among water molecules
H2O(l) + H2O(l) = H3O+(aq) + OH−(aq) Kw = [ H+ ][ OH − ] = 1.0 × 10−14 (at 25°C).
This so-called autoionization reaction occurs only to a very small extent, as shown by the small magnitude of Kw, but this equilibrium forms the basis for the chemistry of aqueous mixtures of acidic and basic solutes. Water acts as a solvent for many polar and ionic species which can then react with water as either an acid, a base, or both. Application of chemical concepts to such aqueous systems, treated according to Brønsted-Lowry formalism, provides an important foundation for biochemistry and physiology. The properties of buffers in aqueous systems fall into this category.