Lecture 2. Atomic theory
Thursday 18 January 2024
Fundamentals of atomic theory. Laws of fixed and multiple proportions. Dalton's atomic theory. Probing subatomic structure. Models for the atom in the early 20th century. Isotopes and the weighted average calculation for atomic mass based on natural isotopic abundance.
Reading: Tro NJ. Chemistry: Structure and Properties (3rd ed.) - Ch.1, §1.1-1.9 (pp.37-60).
Summary
To begin to develop our chemical account of how theories about the nature of atoms and molecules are consistent with and predictive of the properties of substances observed and measured at the scale of our everyday experience, we can follow a historical thread from the 18th century as careful experimental measurements led to the earliest laws of chemistry, then to the first recognizable theory of atoms, right up to the modern form of atomic theory. Along this thread we'll find examples of how the theoretical models for atoms and molecules provide a consistent explanatory framework for experimental data from elements and compounds in bulk.
Development of atomic theory and chemistry as a science in the 18th and 19th centuries
Antoine Lavoisier (1743-1794) who carried out and emphasized the importance of careful measurements, is widely considered the father of chemistry. Lavoisier studied a number of combustion reactions, or more generally what we classify today as oxidation-reduction reactions. Lavoisier, whose education in chemistry included indoctrination in the phlogiston theory, the prevailing concept of these reactions at the time, which held that combustible substances contained a component of fire (a formulation reflecting the persisting influence of the Aristotelian four elements) called phlogiston. When a substance such as wood or charcoal burns, it loses mass, which was "explained" by release of phlogiston from those substances upon burning. This hypothesis was not suited to explain the observation that many substances gained mass upon combustion. With careful and systematic mass measurements of reaction starting material (reactants) and the reaction products, Lavoisier confirmed and extended such results. Furthermore, he clarified the composition of air as a mixture with a component that supported combustion and respiration, and the remainder that could not. To the former he bequeathed the name oxygen. Lavoisier showed that metal oxides (the products of the combustion of the metal) such as those of lead and mercury could be decomposed to the metal and oxygen, and that the masses of reactants and products always remained equal. Lavoisier's results constituted a strong blow to the phlogiston hypothesis, and in its place he offered the hypothesis that in combustion, substances upon burning are combining with oxygen (rather than releasing phlogiston). His published works also included the first clear statement of what would become the Law of the Conservation of Mass.
Atomic number, mass number and isotopes
Musical accompaniment for today's lecture notes.
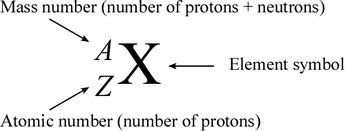
The atomic number of an element (symbolized as Z) is the number of protons in the nuclei of its atoms. The mass number (A) is the total number of nucleons (neutrons and protons). An isotope is made up of atoms of the same element that have the same atomic mass. Different isotopes of an element arise from atoms with differing numbers of neutrons. Because of this, chemists need a way to represent specific isotopes of an element. Isotopes of an element have the same atomic number, but different mass numbers. The atomic number, when represented along with the symbol of an element, is shown as a leading subscript. The mass number is shown as a leading superscript. Since the element symbol implies an atomic number, the latter is often dropped, and an isotope as commonly represented textually with just the mass number and the element symbol (for example 14C or 18O).
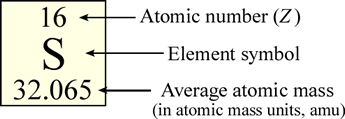
In the periodic table, the elements, represented as their symbols, are arranged in a particular pattern that reflects (as we will see) a regularity, or periodicity in their properties. Typically in the table, the element symbol is contained within its own small box, along with other information including the atomic number and the average atomic mass.
Atoms and ions
In neutral atoms, there are equal numbers of protons and electrons. Elements that exist in nature as isolated, neutral atoms are the exception, rather than the rule. In chemistry, we care most about electrons, and we spend a great deal of time following and counting electrons. Movement of electrons to or from atoms creates charged forms called ions. A positively charged ion is called a cation. A negatively charged ion is called an anion.
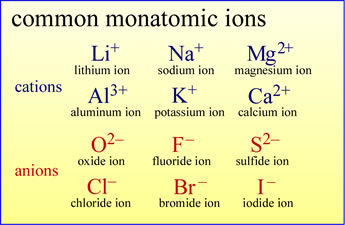
Atoms can lose electrons to become monatomic cations
Atoms can gain electrons to become monatomic anions
The image at left gives the names and symbols of some common monatomic cations and
anions of main group elements.
Note the naming conventions for these ions.
For cations, the ion takes the same name as the element
from which it is derived. For example, the lithium ion is derived from a lithium ion
upon loss of one electron.
In the case of anions, the element name provides a prefix, combined with the suffix "-ide".
As an example, the loss of two electrons from a sulfur atom yields the
"sulf-" + "-ide" = sulfide anion.
Average atomic masses from natural abundances: The weighted-average calculation
The atomic masses given in the periodic table represent weighted averages based on the natural abundances of the isotopes of a given element. The formula for a weighted average (wa) is
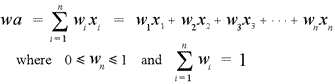
Here the xi's are the masses of the individual isotopes, and the wi's are the fractional abundances corresponding to the isotopes. Note that the weights must sum to 1 (equivalently, the percent abundances must sum to 100%).